Background
Caffeine is the most commonly used nootropic and cognitive-enhancing drug in the world. It is found in nearly every energy drink, pre-workout, tea, and coffee. It is known for antagonizing the adenosine receptor, thus allowing the user to remain alert. However, it also has many other health benefits. Besides the benefits of caffeine, the biggest problem with chronic administration is tolerance buildup. A daily dose of 50-100mg taken for a few weeks no longer elicits the same response, so a new dose of 150-200mg is needed. This tolerance builds up extremely fast, to the point that many people are overconsuming caffeine and turning it into a harmful compound. The truth is, caffeine is wonderful and, when taken correctly, can have tremendous benefits for health and productivity. Fortunately, there are other compounds like caffeine that allow for a caffeine “break” or enhancement.
Caffeine is the most commonly used nootropic and cognitive-enhancing drug in the world. It is found in nearly every energy drink, pre-workout, tea, and coffee. It is known for antagonizing the adenosine receptor, thus allowing the user to remain alert. However, it also has many other health benefits. Besides the benefits of caffeine, the biggest problem with chronic administration is tolerance buildup. A daily dose of 50-100mg taken for a few weeks no longer elicits the same response, so a new dose of 150-200mg is needed. This tolerance builds up extremely fast, to the point that many people are overconsuming caffeine and turning it into a harmful compound. The truth is, caffeine is wonderful and, when taken correctly, can have tremendous benefits for health and productivity. Fortunately, there are other compounds similar to caffeine that allow for a caffeine “break” or enhancement when taken in combination.
One such compound is theacrine, a naturally occurring purine alkaloid found in Camellia Kucha, a common tea whose leaves also produce caffeine at an earlier time point. Caffeine is converted to theacrine via S-adenosyl-l-methionine (SAM) in the leaves of Camellia Kucha. Theacrine acts like caffeine but has much more potential for chronic administration, meaning long-term administration of theacrine does not result in tolerance.
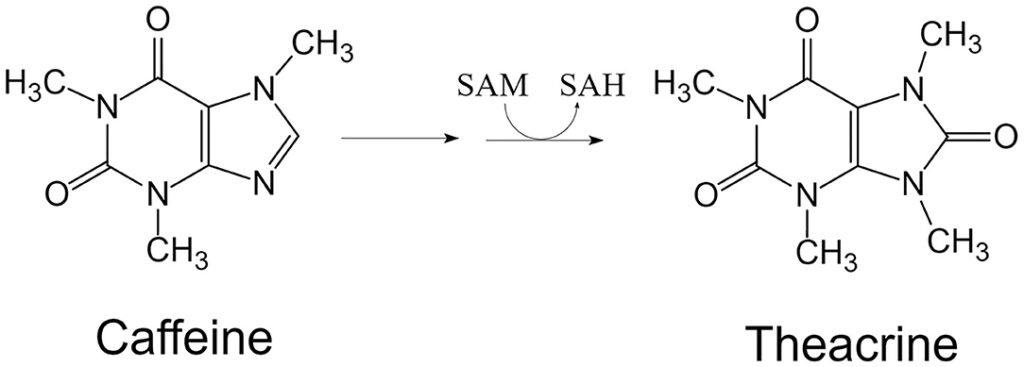
Mechanisms of Action
Theacrine acts through many pathways in the body, although its primary target in eliciting a wakefulness state is the adenosine receptor. Theacrine acts as an adenosine receptor antagonist or blocker, which blocks adenosine from binding and illiciting the signaling cascade that follows. When adenosine builds up, it acts to bind (agonize) the adenosine receptors, which promote drowsiness. By blocking the adenosine receptor, theacrine blocks the ability for adenosine to bind, thus preventing adenosine-induced drowsiness. Theacrine also seems to regulate blood glucose metabolism and restore 5-HTP, a vital molecule in serotonergic signaling. Interestingly, theacrine also restores dopamine levels in certain regions of the brain.
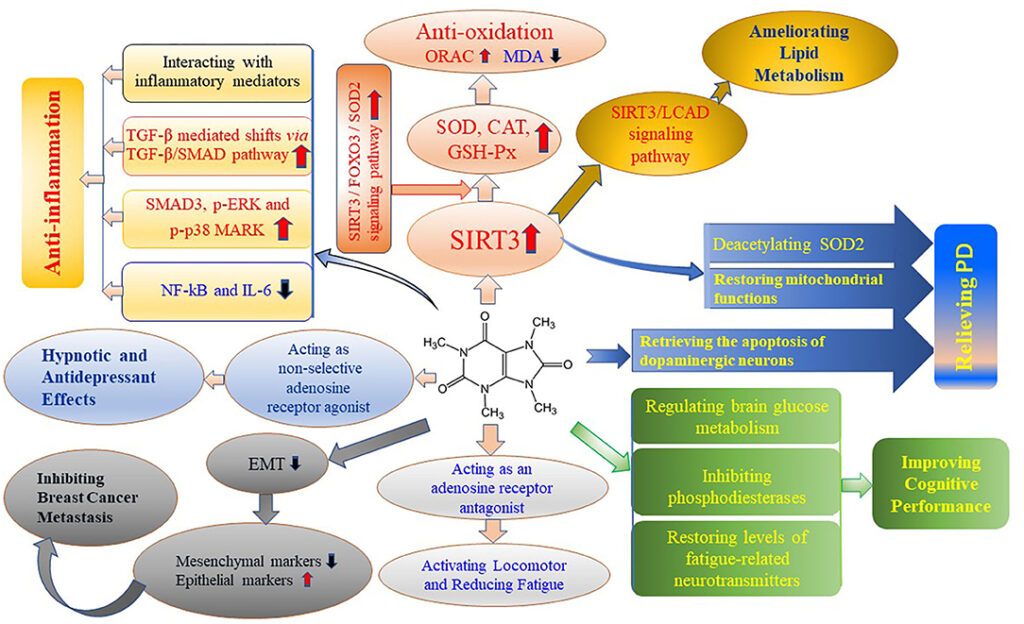
Benefits
Theacrine is a popular compound known as an alternative to caffeine. Although their mechanisms of action are somewhat similar, it is important to note that theacrine does not seem to increase central nervous system excitation to the extent that caffeine does. In terms of half-life, the major advantage of theacrine over caffeine and other stimulants is the long 20-hour half-life. Compared with caffeine’s 5-hour half-life, a one-time dose of theacrine will be sufficient for the entire day.
The primary effect that comes from theacrine administration is wakefulness. This is due to the blocking of the adenosine receptor by theacrine. Theacrine acts as a non-selective adenosine receptor antagonist, thus allowing the user to delay the onset of adenosine-induced drowsiness. This is especially true in the hippocampus, in which researchers measured hippocampal neuron adenosine levels in untreated and theacrine-treated mice. The data show that adenosine levels are significantly higher in the hippocampus of mice treated with theacrine than in the control group, which demonstrates that theacrine blocks adenosine from binding.
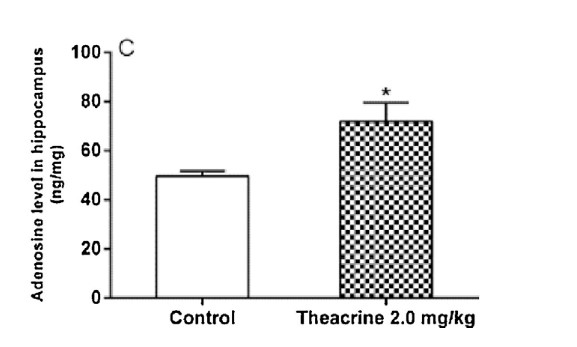
Interestingly, unlike caffeine, which has been known to increase the incidence of insomnia, theacrine has no impact on REM sleep. Additionally, theacrine may be able to alleviate DPCPX-induced insomnia, which may have implications for treating caffeine-induced insomnia. Overall, chronic theacrine intake is used to increase wakefulness while not inducing drug tolerance or insomnia.
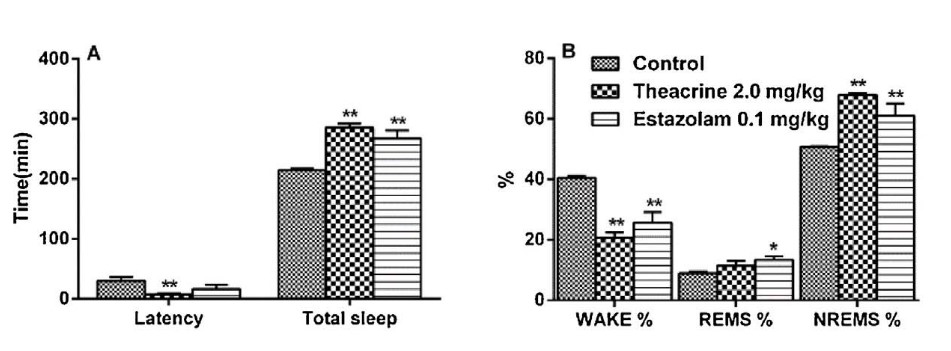
Theacrine also has an interesting role in cognition and inflammation. A paper using soccer players administered theacrine or theacrine plus caffeine to players and compared their performance in a simulated match. The study concluded that theacrine alone or theacrine plus caffeine improved performance, but theacrine plus caffeine was trending towards more improvement compared to theacrine alone. This study indicates that theacrine can enhance performance, but in combination with a low dose of caffeine has a superior impact on performance.
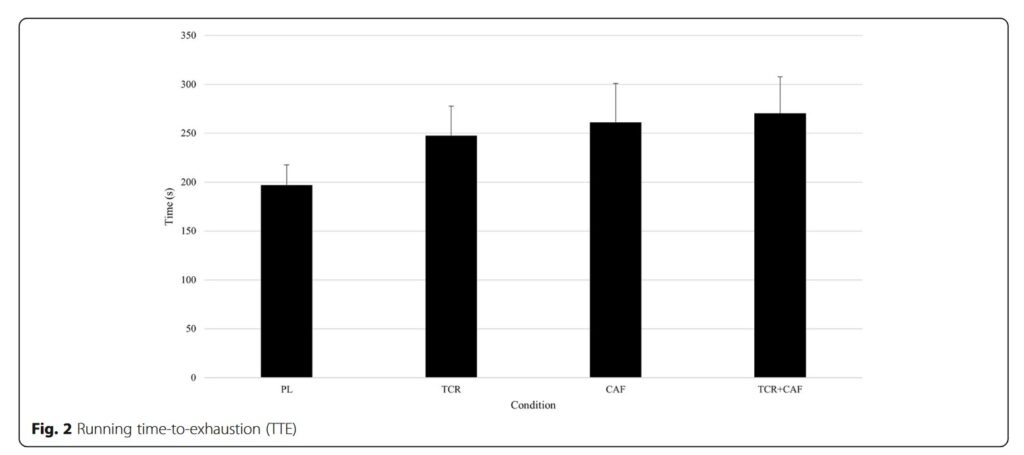
Theacrine’s impact on inflammation is a recent finding in the literature, with only a few studies to support its antioxidant capabilities. One of the first studies exploring theacrine’s antioxidant properties found that theacrine did not act directly to remove free radicals from the cell. Free radicals or reactive oxygen species can increase inflammation by causing damage to the cell, which activates a mechanism in the cell that causes apoptosis. There are several ways to increase these free radicals, such as chronic stress, trauma, or chemical exposure. Theacrine treatment of stressed mice, which induces a pro-inflammatory state, increased the expression of several genes involved in clearing reactive oxygen species and free radicals. The genes that theacrine upregulated were superoxide dismutase, glutathione peroxidase, and catalase, all of which act to decrease free radicals directly. A more recent study found that theacrine is able to activate sirtuin 3 (SIRT3), a member of the sirtuin family. SIRT3 is responsible for regulating apoptosis, or programmed cell death.
- Apoptosis = Programmed cell death that results from cell damage or a signaling message. This cell death can cause inflammation due to the release of intracellular contents into the extracellular space.
SIRT3 regulates apoptosis through superoxide dismutase 2 (SOD2). SOD2 is a mitochondrial dismutase involved in clearing free radicals, thus promoting healthier mitochondrial health and preventing apoptosis. It was shown in the study that theacrine directly activates SIRT3, which protects against apoptotic processes. The big insights come from the fact that theacrine is able to cross the blood-brain barrier, which means the protective properties of theacrine can be facilitated in the brain. A study investigated Parkinson’s-induced mice, which notoriously have a loss of dopaminergic neurons and behavioral issues. The first conclusion the authors made was that theacrine treatment was able to improve behavior in Parkinsonian mice, suggesting the role of theacrine in the dopaminergic system. Next, the authors concluded that theacrine treatment alleviated dopaminergic neuron loss in zebrafish. The importance of this finding is that the death of a neuron is oftentimes a permanent loss due to the slow process of mitosis in neurons. In conclusion, theacrine offers protection from apoptosis through activating SIRT3, which downstream increases free radical scavengers and decreases pro-inflammatory processes.
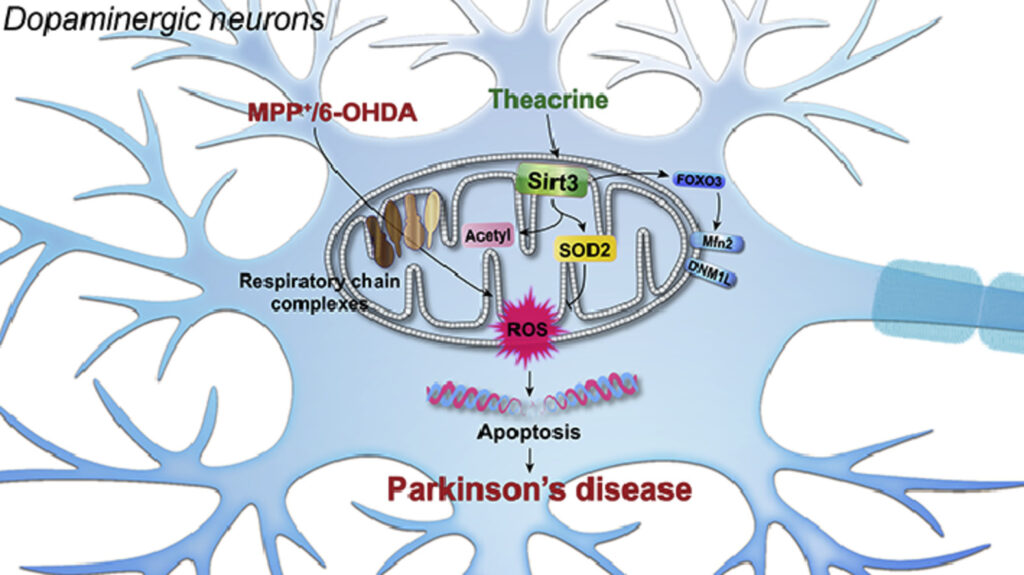
Theacrine is a very useful supplement that has the capability to increase wakefulness, and productivity, act as an anti-inflammatory molecule, and enhance performance. Outside of its benefits, the single most important property of theacrine is that the body does not seem to build a tolerance, which suggests chronic use is safe and effective. This is important because, unlike caffeine, the dose of theacrine can stay the same over time, thus not inducing any toxicity-related side effects. Theacrine is effective at a wide range of doses; perhaps the most commonly used is 0.5–4 mg/kg. For a 170 lb (77 kg) male, an effective dose of theacrine is 100 mg.
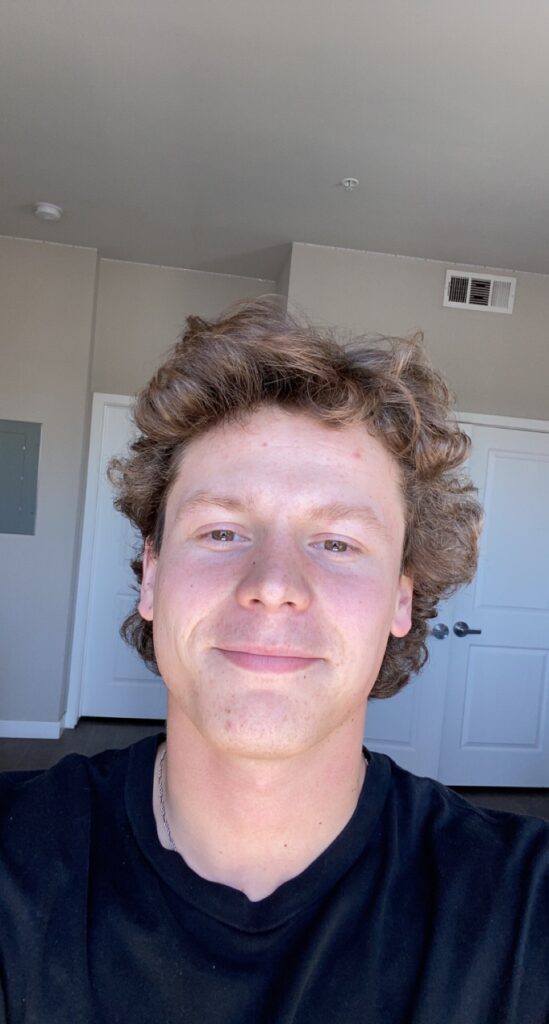
Meet The Author
Hello everyone,
My name is Joshua Giblin. I am a post-bachelor researcher/research technician at USC. My interests range from nutrition to nanomedicine and also practical science to improve everyday life. Through this blog, I aim to communicate practical scientific research and present it to curious individuals so that an educated decision can be made. Thank you for reading the blog and showing your support.
Literature cited
- Bello, M. L., Walker, A. J., McFadden, B. A., Sanders, D. J., & Arent, S. M. (2019). The effects of TeaCrine® and caffeine on endurance and cognitive performance during a simulated match in high-level soccer players. Journal of the International Society of Sports Nutrition, 16(1), 20. https://doi.org/10.1186/s12970-019-0287-6
- Qiao, H., Ye, X., Bai, X., He, J., Li, T., Zhang, J., Zhang, W., & Xu, J. (2017). Theacrine: A purine alkaloid from Camellia assamica var. kucha with a hypnotic property via the adenosine system. Neuroscience Letters, 659, 48–53. https://doi.org/10.1016/j.neulet.2017.08.063
- Sheng, Y.-Y., Xiang, J., Wang, Z.-S., Jin, J., Wang, Y.-Q., Li, Q.-S., Li, D., Fang, Z.-T., Lu, J.-L., Ye, J.-H., Liang, Y.-R., & Zheng, X.-Q. (2020). Theacrine From Camellia kucha and Its Health Beneficial Effects. Frontiers in Nutrition, 7. https://www.frontiersin.org/articles/10.3389/fnut.2020.596823
- Taylor, L., Mumford, P., Roberts, M., Hayward, S., Mullins, J., Urbina, S., & Wilborn, C. (2016). Safety of TeaCrine®, a non-habituating, naturally-occurring purine alkaloid over eight weeks of continuous use. Journal of the International Society of Sports Nutrition, 13(1), 2. https://doi.org/10.1186/s12970-016-0113-3
- Wang, Y., Yang, X., Zheng, X., Li, J., Ye, C., & Song, X. (2010). Theacrine, a purine alkaloid with anti-inflammatory and analgesic activities. Fitoterapia, 81(6), 627–631. https://doi.org/10.1016/j.fitote.2010.03.008
- Ziegenfuss, T. N., Habowski, S. M., Sandrock, J. E., Kedia, A. W., Kerksick, C. M., & Lopez, H. L. (2017). A Two-Part Approach to Examine the Effects of Theacrine (TeaCrine®) Supplementation on Oxygen Consumption, Hemodynamic Responses, and Subjective Measures of Cognitive and Psychometric Parameters. Journal of Dietary Supplements, 14(1), 9–24. https://doi.org/10.1080/19390211.2016.1178678